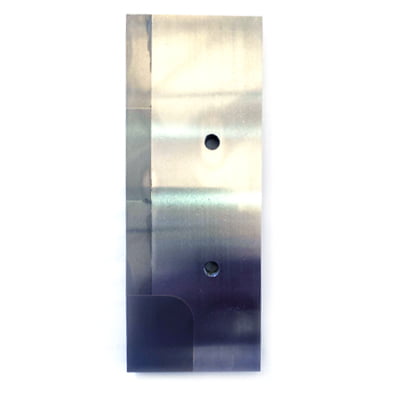
텅스텐 카바이드 용접에 대한 내용
1. 텅스텐 카바이드 브레이징 중 발생하는 문제
텅스텐 카바이드의 납땜성은 상대적으로 낮습니다. 이는 탄소 함량이 높기 때문이며, 세척되지 않은 표면에는 종종 자유 탄소가 포함되어 있어 필러 금속의 젖음을 방해합니다. 또한, 텅스텐 카바이드는 브레이징 온도에서 산화되어 산화물 층을 형성하여 필러 금속의 젖음 특성에 더욱 영향을 미칩니다. 따라서 브레이징 전 표면 세척은 텅스텐 카바이드에서 필러 금속의 젖음성을 향상시키는 데 중요합니다. 필요한 경우, 구리 또는 니켈과 같은 코팅을 브레이징 전에 적용하여 조인트 성능을 개선할 수 있습니다.
2. 브레이징 전 표면 준비
브레이징 전에 작업물 표면의 오염물질을 철저히 제거하는 것이 필수적입니다.산화물, 기름, 먼지, 페인트를 포함한. 적절한 세척 없이는 용융된 충전 금속이 구성 요소 표면을 충분히 적시지 못하고, 조인트 갭을 채울 수도 없습니다. 어떤 경우에는 기본 재료의 브레이징성을 향상시키고 브레이징 조인트의 내식성을 높이기 위해 특정 금속 층으로 부품을 미리 코팅해야 할 수도 있습니다.
(1) 오일 오염물질 제거
오일 오염물질은 알코올, 사염화탄소, 가솔린, 트리클로로에틸렌, 디클로로에탄, 트리클로로에탄과 같은 유기 용매를 사용하여 제거할 수 있습니다. 소량 생산의 경우, 구성 요소를 유기 용매에 담가 철저히 세척할 수 있습니다. 대량 생산에서 가장 일반적인 방법은 증기 탈지입니다. 또한 가열된 알칼리성 용액에서도 효과적인 탈지를 달성할 수 있습니다. 예를 들어, 강철 부품을 70~80°C의 10% 가성소다 용액에 담가둡니다.
(2) 산화물 제거
브레이징 전 부품 표면의 산화물은 기계적 방법, 화학적 에칭 또는 전기 화학적 에칭을 사용하여 제거할 수 있습니다. 기계적 방법에는 파일, 와이어 브러시, 사포, 연삭 휠 및 샌드블라스팅을 사용하여 산화막을 제거할 수 있습니다. 파일과 사포는 일반적으로 단일 부품 생산에 사용되며, 필러 금속의 습윤 및 확산에 유리한 홈을 만듭니다. 일괄 생산의 경우 샌더, 와이어 브러시 및 샌드블라스팅 방법이 더 효율적입니다. 기계적 세척 방법은 알루미늄 합금 및 티타늄 합금에 적합하지 않습니다.
(3) 금속으로 코팅된 기본 소재
기본 재료 표면에 금속 코팅을 적용하는 것은 주로 특정 재료의 브레이징성을 개선하고 필러 금속의 습윤성을 향상시키는 것을 목표로 합니다. 이 공정은 또한 균열 형성이나 취성 금속간 화합물의 발달과 같이 접합 품질을 저하시킬 수 있는 기본 재료와 필러 금속 간의 부정적인 상호 작용을 방지할 수 있습니다. 또한 조립을 단순화하고 생산성을 개선하기 위한 필러 층 역할을 할 수도 있습니다.
3. 브레이징 재료
(1) 필러 메탈
일반적인 필러 금속에는 순수 구리, 구리-아연 합금 및 은-구리 합금이 있습니다. 순수 구리는 다양한 텅스텐 카바이드 재료와 우수한 습윤성을 제공하지만 최적의 결과를 얻으려면 수소 환원 분위기에서 브레이징해야 합니다. 그러나 브레이징 온도가 높기 때문에 조인트에서 발생하는 열 응력으로 인해 균열 가능성이 높아질 수 있습니다. 기존의 순수 구리 브레이징 조인트는 전단 강도가 약 150MPa이고 연성이 상당히 높지만 고온 응용 분야에는 적합하지 않습니다.
구리-아연 필러는 텅스텐 카바이드 및 공구강의 브레이징에 가장 널리 사용됩니다. 젖음성과 접합 강도를 향상시키기 위해 Mn, Ni 및 Fe와 같은 합금 원소가 종종 추가됩니다. 예를 들어, 약 4 wt%의 망간 함량을 갖는 B-Cu58ZnMn에서 텅스텐 카바이드 브레이징 접합부의 전단 강도는 실온에서 300~320MPa에 도달할 수 있으며 320°C에서 220~240MPa를 유지합니다. B-Cu58ZnMn에 소량의 코발트를 추가하면 전단 강도를 350MPa까지 높이는 동시에 더 높은 충격 인성과 피로 저항성을 제공하여 서비스 수명을 크게 연장할 수 있습니다. 절단 도구 및 록 드릴 비트.
은-구리 필러는 녹는점이 낮아 텅스텐 카바이드 브레이징 중 열 응력이 최소화되고 균열 경향이 낮아집니다. 젖음성을 개선하고 조인트 강도와 작동 온도를 높이기 위해 Mn 및 Ni와 같은 합금 원소가 일반적으로 통합됩니다. 예를 들어, B-Ag50CuZnCdNi 필러는 텅스텐 카바이드와 우수한 젖음성을 나타내며 브레이징 조인트에 바람직한 전반적인 성능을 제공합니다.
500°C 이상에서 높은 접합 강도가 요구되는 응용 분야의 경우, B-Mn50NiCuCrCo 및 B-Ni75CrSiB와 같은 망간 기반 및 니켈 기반 필러를 선택할 수 있습니다. 고속강을 브레이징할 때는 담금질 온도에 맞는 특정 필러 재료가 필요하며, 이는 두 가지 범주로 나뉩니다. 망간-철 기반 필러는 주로 망간 철과 붕사로 구성되어 일반적으로 약 100MPa의 전단 강도를 제공하지만 이러한 접합은 균열이 발생하기 쉽습니다. 다른 범주는 Ni, Fe, Mn 및 Si를 함유한 특수 구리 합금으로 구성되어 균열 경향을 최소화하고 최대 300MPa의 전단 강도를 달성합니다.
(2) 플럭스 및 보호 가스
플럭스 선택은 기본 소재와 선택한 필러 금속 모두와 일치해야 합니다. 브레이징 도구강과 텅스텐 카바이드의 경우, 주로 사용되는 플럭스는 붕사와 붕산이며, 종종 다양한 불화물(KF, NaF, CaF2 등)로 보충됩니다. 구리-아연 필러는 일반적으로 FB301, FB302 및 FB105 플럭스와 함께 사용되는 반면, 은-구리 필러는 FB101~FB104 플럭스를 활용할 수 있습니다. 고속강 브레이징에 특수 필러를 사용하는 경우, 주로 붕사 플럭스가 사용됩니다.
가열 공정 중 공구강의 산화를 방지하고 브레이징 후 세척을 없애기 위해 가스 차폐 브레이징을 사용할 수 있습니다. 보호 가스는 불활성 가스 또는 환원 가스일 수 있으며, 가스 이슬점은 -40°C 미만이어야 합니다. 텅스텐 카바이드는 수소 보호 하에 브레이징할 수 있으며, 수소 이슬점은 -59°C 미만이어야 합니다.
4. 브레이징 공정
탄소 공구강을 브레이징하려면 표면을 주의 깊게 청소해야 하며, 기계적 표면은 지나치게 매끈할 필요가 없습니다. 이는 재료와 플럭스의 습윤 및 확산을 돕기 때문입니다. 텅스텐 카바이드의 표면은 일반적으로 실리콘 카바이드 또는 다이아몬드 휠로 사포 분사 또는 연삭하여 필러 금속에 의한 과도한 탄소를 제거하여 더 나은 습윤을 제공해야 합니다. 카바이드-티타늄 텅스텐 카바이드는 습윤에 어려움이 있습니다. 따라서 표면에 구리 또는 니켈 산화물 페이스트를 바르고 환원 분위기에서 가열하면 효과적인 습윤성이 촉진됩니다.
탄소 공구강 브레이징은 담금질 전이나 이 공정과 동시에 수행하는 것이 가장 좋습니다. 담금질 전에 브레이징을 수행하는 경우 필러 메탈의 고상선 온도는 담금질 온도 범위를 초과해야 하며, 접합된 조각이 담금질 온도로 재가열될 때 충분한 강도를 유지하도록 해야 합니다. 브레이징과 담금을 동시에 수행하는 경우 고상선 온도가 담금질 온도에 가까운 필러 메탈을 선택해야 합니다.
합금 공구강의 구성 범위는 광범위하므로 최적의 접합 성능을 달성하기 위해 특정 강철 유형에 따라 특정 충전 금속 및 열처리 공정 선택이 필요합니다.
고속강의 경우, 담금질 온도는 일반적으로 은-구리 및 구리-아연 필러의 용융 온도를 초과하여 사전 담금질이 필요합니다. 브레이징은 2차 템퍼링 중 또는 그 후에 수행할 수 있습니다. 브레이징 후 담금질이 필요한 경우 앞서 언급한 특수 필러만 사용해야 합니다. 고속강 공구를 브레이징할 때 코크스로를 사용하면 매우 효과적일 수 있습니다. 필러가 녹은 후 절삭 공구를 제거하고 과도한 필러를 눌러낸 다음 오일 담금질을 거친 후 550~570°C에서 템퍼링을 실시해야 합니다.
텅스텐 카바이드 블레이드를 강철 공구 본체에 납땜할 때는 납땜 이음매의 간격을 늘리고 플라스틱 보상 틈을 추가하는 방법과 용접 후 느린 냉각을 통해 열 응력을 줄이고 균열 형성을 방지하는 방법을 사용해야 하며, 이를 통해 텅스텐 카바이드 절삭 공구 조립체의 수명을 연장해야 합니다.
5. 브레이징 후 세척
플럭스에서 나온 잔류물은 브레이징된 조인트를 부식시킬 수 있으며 조인트 검사를 방해할 수 있으므로 철저히 제거해야 합니다. 처음에는 작업물에 남은 플럭스를 뜨거운 물이나 일반적인 플럭스 제거 혼합물로 헹군 다음 적절한 산 세척 용액으로 처리하여 기본 재료에서 산화물 층을 제거할 수 있습니다. 브레이징된 금속의 부식을 방지하기 위해 질산 용액을 사용하지 않는 것이 중요합니다. 유기 연성 플럭스에서 나온 잔류물은 가솔린, 알코올 또는 아세톤과 같은 용매로 세척할 수 있으며, 산화 아연 및 염화 암모늄과 같은 부식성 잔류물은 10% NaOH 용액으로 세척한 다음 뜨거운 물이나 차가운 물로 헹구어야 합니다. 붕사와 붕산 플럭스에서 나온 잔류물은 일반적으로 기계적 방법이나 물에서 장시간 끓여서 분해할 수 있습니다.
6. 브레이징 품질 검사
브레이징 접합부를 검사하는 방법은 비파괴 검사와 파괴 검사로 나눌 수 있습니다. 다음은 비파괴 검사 방법을 강조합니다.
- 시각 검사
- 염색 침투 및 형광 테스트: 이러한 방법은 주로 시각적 검사를 통해 볼 수 없는 작은 균열, 기공 또는 기타 결함을 감지하는 데 사용됩니다.
이 포괄적인 분석은 텅스텐 카바이드 브레이징의 중요한 문제와 해결책을 강조하여 전반적인 조인트 성능과 품질을 이해하고 향상시키는 데 도움이 됩니다.